During the lifetime of a star a balance is maintained between the outward pressure of fusion and the inward force of gravity. The duration of this stable period (which in the case of the sun is about 10 billion years) will depend on the mass of the star: the bigger the mass the shorter its life will be. In the case of our Sun it will expand towards the end of its life, leaving the main sequence to become a red giant, absorbing the inner planets as it does so. At the end of this expansion phase the radiation will decrease and the star will begin to collapse into a white dwarf.
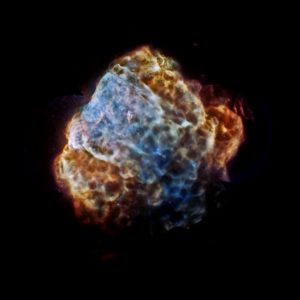
For the more massive stars their end point is more energetic, more dramatic. Because of the greater mass the pressure on the core will be greater so making the temperatures much higher. This will make fusion take place at a faster rate so the time in the main sequence will be relatively shorter. A star ten times more massive than the sun, for instance, will consume its hydrogen a thousand times faster.
The fusion in the red giant phase will last about half a million years as three helium nuclei fuse to form carbon. The radiation from this process will prevent the star from collapsing into a white dwarf. Nevertheless the red giant will eventually collapse when the next phase begins as its carbon fuses to oxygen. The relentless pressure of gravity will by degrees form layers of neon-20, magnesium-24, silicon-28, sulphur-32, argon-40, calcium-40 and titanium-48. By the time silicon is formed elements begin to fuse into iron-56 at the core and the entire star could well be described as having shells like the skins of an onion. At this stage there is simply not enough energy to carry the process beyond iron.
There follows a catastrophic final collapse under extreme gravitational forces. Because the core cannot compress any further this creates a backlash that rips the star apart. The speed of expansion can reach around 10 000 kilometres a second—displayed as a supernova. All that is left is a small core of neutrons, a neutron star. Its size will depend on the original mass of the star, anything from just two kilometres to twenty kilometres in diameter—that’s right, as small as two kilometres across. The average for a neutron star is thought to be around 15 kilometres in diameter and some 1.4 times the mass of our sun. You could think of a neutron star as a gigantic atomic nucleus. A tablespoon of the material would weigh about 900 billion kilogrammes.
End of life behaviour | |
---|---|
Solar mass | |
<0.5 | Star uses up its hydrogen and then collapses to a white dwarf and finally a black dwarf |
0.5 - 1.4 | Once the star uses up its hydrogen it expands to a red giant. It loses energy and shrinks down to a white dwarf, and finally to a black dwarf |
1.5 - 3.0 | Supergiant explodes in a supernova collapsing to a Neutron star |
>3.0 | Supergiant explodes in a supernova collapsing to a Black hole |
Note: > more than; < less than |
The Chandrasekhar limit
As we have mentioned the path a star follows to destruction depends on its mass. And a very clever astrophysicist worked out the appropriate signpost at the fork in the road. His name was Subrahmanyan Chandrasekhar (1910 – 1995) of the University of Chicago where in the 1950s he established the so-called “Chandrasekhar limit”.
He found that when matter is compressed there is a limit to the process where electrons surrounding a nuclei offer resistance because they are negatively charged. “Like charges” repel each other, and you can only push them together so far beyond which you can go no further. This balance is reached and can be maintained if the mass of the star is less than 1.44 solar masses. Thus, 1.44 is the Chandrasekhar limit.
A white dwarf star of less than 1.44 solar masses can go on existing indefinitely. The only time such a white dwarf can be demolished is:
1] at the carbon-oxygen stage, or
2] if it is a companion in a close binary.
The second instance is a common configuration in the universe. Here the white dwarf gradually strips matter away from its big brother because of the dwarf’s highly concentrated gravity. The dwarf will increase in size reaching beyond the 1.44 mass limit. The increased pressure on the core will raise the temperature so that fusion will recommence. When the energy flux exceeds 20 times the binding energy of the star it will blow itself to smithereens, with the outer layers shooting off at a rate of 10 000 kilometres a second. During the explosion at least one solar mass of the unstable nickel-56 is formed. This decays to cobalt-56 and finally to iron-56.
In the case of this white dwarf the speed of decay is commensurate with the fading of a Type I supernova. Apparently no remnant is left when a white dwarf goes supernova.
Back to Top
By Nigel Benetton, science fiction author of Red Moon Burning and The Wild Sands of Rotar.
Last updated:Saturday, 20 March 2021